ジペプチジルペプチダーゼ-4阻害剤の発見と開発
Discovery and development of dipeptidyl peptidase-4 inhibitors/ja
ジペプチジルペプチダーゼ-4阻害薬(DPP-4阻害薬)は、酵素ジペプチジルペプチダーゼ-4 (DPP-4)を阻害する酵素阻害薬である。これらは2型糖尿病の治療に用いられる。DPP-4酵素の阻害は、インスリン分泌と血糖コントロール調節に重要な役割を果たすインクレチンの活性を延長・増強する。 2型糖尿病は、膵臓のβ-cellが身体の必要量を満たすのに十分な量のインスリンを分泌できないことから生じる慢性の代謝性疾患である。インスリン抵抗性および肝グルコース産生の増加もまた、インスリンに対する身体の需要を増加させることによって役割を果たすことがある。インスリン補充以外の現在の治療法は、時にコントロールを達成するのに十分ではなく、体重増加や低血糖などの望ましくない副作用を引き起こすことがある。近年、インスリンの産生機構や体内の糖代謝の調節機構に関する継続的な研究に基づいて、新しい薬物が開発されている。DPP-4という酵素が重要な役割を果たしていることがわかってきた。
歴史
1967年に発見されて以来、セリンプロテアーゼDPP-4は人気のある研究対象であった。DPP-4の阻害剤は、酵素の機能的意義を解明するためのツールとして長い間求められてきた。最初の阻害剤は1980年代後半から1990年代にかけて特徴づけられた。それぞれの阻害剤は、その後の研究のための初期の構造活性相関(SAR)を確立するために重要であった。阻害剤は、DPP-4と共有結合で相互作用するものとそうでないものの2つの主要なクラスに分類される。DPP-4はP1位にプロリンを含む基質と選択的に結合するジペプチダーゼであるため、多くのDPP-4阻害剤はプロリンを模倣した5員複素環を持つ。例えば、ピロリジン、シアノピロリジン、チアゾリジン、シアノチアゾリジンなどである。これらの化合物は一般的に触媒残基Ser630と共有結合を形成する。
1994年、ゼリア新薬の研究者はニトリルを官能基とするシアノピロリジンを発表した。これは触媒であるセリンとイミデートを形成すると想定されていた。同時に、ニトリル基を持たない他のDPP-4阻害剤も発表されたが、それらは他のセリンと相互作用するモチーフ、例えばボロン酸、ホスホン酸またはジアシルヒドロキシルアミンを含んでいた。これらの化合物は、DPP-4とプロリルオリゴペプチダーゼ(PEP)が類似しているために強力ではなく、また化学的不安定性に悩まされていた。フェリング製薬は2つのシアノピロリジンDPP-4阻害剤の特許を申請し、1995年に公開した。これらの化合物は優れた効力を持ち、化学的安定性も改善されていた。
1995年、Novartisのエドウィン・B・ヴィルハウアーは、DPP-4がN-メチルグリシンをN-末端のアミノ酸として同定するという事実に基づいて、N-置換グリシニル-シアノピロリジンの探索を開始した。この新しいシアノピロリジン群は、その後の数年間、非常に人気のある研究分野となった。バソペプチダーゼ阻害はインスリン分泌を刺激することによってDPP-4阻害薬の抗糖尿病効果を増強すると考えられているからである。バソペプチダーゼ阻害モチーフはDPP-4阻害剤とN-置換基でつながっている。
DPP-4のメカニズム
図1:'食事中に、インクレチンであるグルカゴン様ペプチド1(GLP-1)とグルコース依存性胃抑制ポリペプチド(GIP)が小腸から血液中に放出される。これらのホルモンはグルコース依存的にインスリン分泌を調節する。(GLP-1は人体において多くの役割を持っている。インスリンの生合成を刺激し、グルカゴンの分泌を抑制し、胃排出を遅らせ、食欲を減退させ、膵島β-cellの再生を刺激する)
GLP-1とGIPは、酵素DPP-4によって触媒される非常に速い不活性化により、血漿半減期が極めて短い。DPP-4である。DPP-4を阻害すると、これらの不活性化が遅くなり、作用が増強され、血漿中のグルコース濃度が低下するので、2型糖尿病の治療に有用である。(図1)。
DPP-4の分布と機能
DPP-4は身体のほとんど全ての臓器の内皮の細胞膜に付着している。DPP-4を強く発現している組織には、外分泌膵臓、汗腺、唾液腺および乳腺、胸腺, リンパ節、胆道、腎臓、肝臓、胎盤、子宮、前立腺、皮膚、腸粘膜の毛細血管床などがある。(ここでほとんどのGLP-1は局所的に不活性化される)。また、血漿や脳脊髄液などの体液にも可溶性で存在する(DPP-4はCD26T細胞を活性化する抗原である)。
DPP-4は、GLP-1やGIPなどのペプチドから、2位にプロリンやアラニンを持つ2つのアミノ酸を選択的に切断する(図2)。DPP-4が効果を発揮する活性部位には、Asp-His-Serの3つのアミノ酸が特徴的に配置されている。 GPL-1やGIPの生物活性にはアラニンとプロリンが重要であるため、これらのアミノ酸を切断することで不活性化される。したがって、インクレチンホルモンの分解を防ぐことができる。分解防止は、2型糖尿病の治療戦略としての可能性を秘めている。
DPP-4の特徴
DPP-4はプロテアーゼであるため、阻害剤がペプチドの性質を持つことは予想外ではなく、このテーマは現代の研究にも受け継がれている。
構造
2003年以降に発表されたDPP-4のX線構造は、結合部位の構造的特徴についてかなり詳細な情報を与えている。多くの構造的に多様なDPP-4阻害剤が発見されており、結合部位の特性を考えればそれほど驚くことではない:
1. A deep lipophilic pocket combined with several exposed aromatic side chains for achieving high affinity small molecule binding.
2. A significant solvent access that makes it possible to tune the physico-chemical properties of the inhibitors that leads to better pharmacokinetic behavior.
DPP-4 is a 766-amino acid transmembrane glycoprotein that belongs to the prolyloligopeptidase family. It consists of three parts; a cytoplasmic tail, a transmembrane region and an extracellular part. The extracellular part is divided into a catalytic domain and an eight-bladed β-propeller domain. The latter contributes to the inhibitor binding site. The catalytic domain shows an α/β-hydrolase fold and contains the catalytic triad Ser630 - Asp708 - His740. The S1-pocket is very hydrophobic and is composed of the side chains: Tyr631, Val656, Trp662, Tyr666 and Val711. Existing X-ray structures show that there is not much difference in size and shape of the pocket that indicates that the S1-pocket has high specificity for proline residues
Binding site

DPP-4 inhibitors usually have an electrophilic group that can interact with the hydroxyl of the catalytic serine in the active binding site (Figure 3). Frequently that group is a nitrile group but can also be boronic acid or diphenyl phosphonate. This electrophilic group can bind to the imidate complex with covalent bonds and slow, tight-binding kinetics but this group is also responsible for stability issues due to reactions with the free amino group of the P2-amino acid. Therefore, inhibitors without the electrophilic group have also been developed, but these molecules have shown toxicity due to affinity to other dipeptidyl peptidases, e.g. DPP-2, DPP-8 and DPP-9.
DPP-4 inhibitors span diverse structural types. In 2007 few of the most potent compounds contain a proline mimetic cyanopyrrolidine P1 group. This group enhances the potency, probably due to a transient covalent trapping of the nitrile group by the active site Ser630 hydroxyl, leading to delayed dissociation and slow tight binding of certain inhibitors. When these potency enhancements were achieved, some chemical stability issues were noted and more advanced molecules had to be made. To avoid these stability issues, the possibility to exclude the nitrile group was investigated. Amino acids with aryl or polar side chains did not show appreciable DPP-4 inhibition and in fact, all compounds without the nitrile group in this research suffered a 20 to 50-fold loss of potency corresponding to the compounds containing the nitrile group.
Discovery and development
It is important to find a fast and accurate system to discover new DPP-4 inhibitors with ideal therapeutic profiles. High throughput screening (HTS) usually gives low hit rates in identifying the inhibitors but virtual screening (VS) can give higher rates. VS has for example been used to screen for small primary aliphatic amines to identify fragments that could be placed in S1 and S2 sites of DPP-4. On the other hand, these fragments were not very potent and therefore identified as a starting point to design better ones. Three-dimensional models can provide a useful tool for designing novel DPP-4 inhibitors. Pharmacophore models have been made based on key chemical features of compounds with DPP-4 inhibitory activity. These models can provide a hypothetical picture of the primary chemical feature responsible for inhibitory activity. The first DPP-4 inhibitors were reversible inhibitors and came with bad side effects because of low selectivity. Researchers suspected that inhibitors with short half-lives would be preferred in order to minimize possible side effects. However, since clinical trials showed the opposite, the latest DPP-4 inhibitors have a long-lasting effect. One of the first reported DPP-4 inhibitor was P32/98 from Merck. It used thiazolidide as the P1-substitute and was the first DPP-4 inhibitor that showed effects in both animals and humans but it was not developed to a market drug due to side effects. Another old inhibitor is DPP-728 from Novartis, where 2-cyanopyrrolidine is used as the P1-substitute. The addition of the cyano group generally increases the potency. Therefore, researchers' attention was directed to those compounds. Usually, DPP-4 inhibitors are either substrate-like or non-substrate-like.

Substrate-like inhibitors
Substrate-like inhibitors (Figure 4) are more common than the non-substrate-likes. They bind either covalently or non-covalently and have a basic structure where the P1-substituent occupies the S1-pocket and the P2-substituent occupies the S2-pocket. Usually they contain a proline mimetic that occupies the S1-pocket. Large substituents on the 2-cyanopyrrolidine ring are normally not tolerated since the S1-pocket is quite small. Since DPP-4 is identical with the T-cell activation marker CD26 and DPP-4 inhibitors are known to inhibit T-cell proliferation, these compounds were initially thought to be potential immunomodulators. When the function against type 2 diabetes was discovered, the cyanopyrrolidines became a highly popular research material. A little later vildagliptin and saxagliptin, which are the most developed cyanopyrrolidine DPP-4 inhibitors to date, were discovered.
Cyanopyrrolidines
Cyanopyrrolidines have two key interactions to the DPP-4 complex:
1. Nitrile in the position of the scissile bond of the peptidic substrate that is important for high potency. The nitrile group forms reversible covalent bonds with the catalytically active serine hydroxyl (Ser630), i.e. cyanopyrrolidines are competitive inhibitors with slow dissociation kinetics.
2. Hydrogen bonding network between the protonated amino group and a negatively charged region of the protein surface, Glu205, Glu206 and Tyr662. All cyanopyrrolidines have basic, primary or secondary amine, which makes this network possible but these compounds usually drop in potency if these amines are changed. Nonetheless, two patent applications unveil that the amino group can be changed, i.e. replaced by a hydrazine, but it is claimed that these compounds do not only act via DPP-4 inhibition but also prevent diabetic vascular complications by acting as a radical scavenger.
Structure-activity relationship (SAR)
Important structure-activity relationship:
1. Strict steric constraint exists around the pyrrolidine ring of cyanopyrrolidine-based inhibitors, with only hydrogen, fluoro, acetylene, nitrile, or methano substitution permitted.
2. Presence of a nitrile moiety on the pyrrolidine ring is critical to achieving potent activity
Also, systematic SAR investigation has shown that the ring size and stereochemistry for the P2 position is quite conditioned. A 5-membered ring and L-configuration has shown better results than a 4-membered or 6-membered ring with D-configuration. Only minor changes on the pyrrolidine ring can be tolerated, since the good fit of the ring with the hydrophobic S1 pocket is very important for high affinity. Some trials have been made, e.g. by replacing the pyrrolidine with a thiazoline. That led to improved potency but also loss of chemical stability. Efforts to improve chemical stability often led to loss of specificity because of interactions with DPP-8 and DPP-9. These interactions have been connected with increased toxicity and mortality in animals. There are strict limitations in the P1 position and hardly any changes are tolerated. On the other hand, a variety of changes can be made in the P2 position. In fact, substitution with quite big branched side chains, e.g. tert-butylglycin, normally increased activity and chemical stability, which could lead to longer-lasting inhibition of the DPP-4 enzyme. It has also been noted that biaryl-based side chains can also give highly active inhibitors. It was originally believed that only lipophilic substitution would be tolerated. Now it is stated that also the substitution of polar negatively charged side-chains as well as hydrophilic substitution can lead to excellent inhibitory activity.
Chemical stability

In general, DPP-4 inhibitors are not very stable compounds. Therefore, many researchers focus on enhancing the stability for cyanopyrrolidines. The most widespread technique to improve chemical stability is to incorporate a steric bulk. The two cyanopyrrolidines that have been most pronounced, vildagliptin and saxagliptin, were created in this manner. K579 is a DPP-4 inhibitor discovered by researchers at Kyowa Hakko Kyogo. It had improved not only chemical stability but also a longer-lasting action. That long-lasting action was most likely due to slow dissociation of the enzyme-inhibitor complex and an active oxide metabolite that undergoes enterohepatic circulation. The discovery of the active oxide was in fact a big breakthrough as it led to the development of vildagliptin and saxagliptin. One major problem in DPP-4 inhibitor stability is intramolecular cyclization. The precondition for the intramolecular cyclization is the conversion of the trans-rotamer, which is the DPP-4 binding rotamer (Figure 5). Thus, preventing this conversion will increase stability. This prevention was successful when incorporating an amide group into a ring, creating a compound that kept the DPP-4 inhibitory activity that, did not undergo the intramolecular cyclization and was even more selective over different DPP enzymes. It has also been reported that a cyanoazetidine in the P1 position and a β-amino acid in the P2 position increased stability.
Vildagliptin
Vildagliptin (Galvus)(Figure 6) was first synthesized in May 1998 and was named after Edwin B. Villhauer. It was discovered when researchers at Novartis examined adamantyl derivatives that had proven to be very potent. The adamantyl group worked as a steric bulk and slowed intramolecular cyclization while increasing chemical stability. Furthermore, the primary metabolites were highly active. To avoid additional chiral center a hydroxylation at the adamantyl ring was carried out (Figure 6). The product, vildagliptin, was even more stable, undergoing intramolecular cyclization 30-times slower, and having high DPP-4 inhibitory activity and longer-lasting pharmacodynamic effect.
Saxagliptin

Researchers at Bristol-Myers Squibb found that increased steric bulk of the N-terminal amino acid side-chain led to increased stability. To additionally increase stability the trans-rotamer was stabilized with a cis-4,5-methano substitution of the pyrrolidine ring, resulting in an intramolecular van-der-Waals interaction, thus preventing intramolecular cyclisation. Because of that increased stability, the researchers continued their investigation on cis-4,5-methano cyanopyrrolidines and came across with a new adamantyl derivative, which showed extraordinary ex vivo DPP-4 inhibition in rat plasma. Also noted, high microsomal turnover rate which indicated that the derivative was quickly converted to an active metabolite. After hydroxylation on the adamantyl group they had a product with better microsomal stability and improved chemical stability. That product was named saxagliptin (Onglyza) (Figure 6). In June 2008 AstraZeneca and Bristol-Myers Squibb submitted a new drug application for Onglyza in the United States and a marketing authorization application in Europe. Approval was granted in the United States by the FDA in July 2009 for Onglyza 5 mg and Onglyza 2.5 mg. This was later combined with extended-release metformin (taken once daily) and approved by the FDA in January 2011 under the trade name Kombiglyze XR.
Denagliptin
Denagliptin (Figure 6) is an advanced compound with a branched side-chain at the P2 position, but also has (4S)-fluoro substitution on the cyanopyrrolidine ring. It is a well-known DPP-4 inhibitor developed by GlaxoSmithKline (GSK). Biological evaluations have shown that the S-configuration of the amino acid portion is essential for the inhibitory activity since the R-configuration showed reluctantly inhibition. These findings will be useful in future designs and synthesis of DPP-4 inhibitors. GSK suspended Phase III clinical trials in October 2008.
Azetidine based compounds
Informations for this group of inhibitors are quite restricted. Azetidine-based DPP-4 inhibitors can roughly be grouped into three main subcategories: 2-cyanoazetidines, 3-fluoroazetidines, and 2-ketoazetidines. The most potent ketoazetidines and cyanoazetidines have large hydrophobic amino acid groups bound to the azetidine nitrogen and are active below 100nM.
Non-substrate-like inhibitors
Non-substrate-like inhibitors do not take after dipeptidic nature of DPP-4 substrates. They are non-covalent inhibitors and usually have an aromatic ring that occupies the S1-pocket, instead of the proline mimetic.
In 1999, Merck started a drug development program on DPP-4 inhibitors. When they started internal screening and medicinal chemistry program, two DPP-4 inhibitors were already in clinical trials, isoleucyl thiazolidide (P32/38) and NVP-DPP728 from Novartis. Merck in-licensed L-threo-isoleucyl thiazolidide and its allo stereoisomer. In animal studies, they found that both isomers had similar affinity for DPP-4, similar in vivo efficacy, similar pharmacokinetic and metabolic profiles. Nevertheless, the allo isomer was 10-fold more toxic. The researchers found out that this difference in toxicity was due to the allo isomer's greater inhibition of DPP-8 and DPP-9 but not because of selective DPP-4 inhibition. More research also supported that DPP-4 inhibition would not cause compromised immune function. Once this link between affinity for DPP-8/DPP-9 and toxicity was discovered, Merck decided on identifying an inhibitor with more than a thousandfold affinity for DPP-4 over the other dipeptidases. For this purpose, they used positional scanning libraries. From scanning these libraries, the researchers discovered that both DPP-4 and DPP-8 showed a strong preference for breaking down peptides with a proline at the P1 position but they found a great difference at the P2 site; i.e., they found that acidic functionality at the P2 position could provide a greater affinity for DPP-4 over DPP-8. Merck kept up doing even more research and screening. They stopped working on compounds from the α-amino acid series related to isoleucyl thiazolidide due to lack of selectivity but instead they discovered a very selective β-amino acid piperazine series through SAR studies on two screening leads. When trying to stabilize the piperazine moiety, a group of bicyclic derivatives were made, which led to the identification of a potent and selective triazolopiperazine series. Most of these analogs showed excellent pharmacokinetic properties in preclinical species. Optimization of these compounds finally led to the discovery of sitagliptin.
Sitagliptin
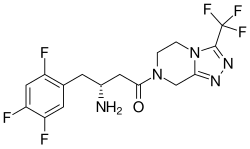
Sitagliptin (Januvia) has a novel structure with β-amino amide derivatives (Figure 7). Since sitagliptin has shown excellent selectivity and in vivo efficacy it urged researchers to inspect the new structure of DPP-4 inhibitors with appended β-amino acid moiety. Further studies are being developed to optimize these compounds for the treatment of diabetes. In October 2006 sitagliptin became the first DPP-4 inhibitor that got FDA approval for the treatment of type 2 diabetes. Crystallographic structure of sitagliptin along with molecular modeling has been used to continue the search for structurally diverse inhibitors. A new potent, selective and orally bioavailable DPP-4 inhibitor was discovered by replacing the central cyclohexylamine in sitagliptin with 3-aminopiperidine. A 2-pyridyl substitution was the initial SAR breakthrough since that group plays a significant role in potency and selectivity for DPP-4.
It has been shown with an X-ray crystallography how sitagliptin binds to the DPP-4 complex:
1. The trifluorophenyl group occupies the S1-pocket
2. The trifluoromethyl group interacts with the side chains of residues Arg358 and Ser209.
3. The amino group forms a salt bridge with Tyr662 and the carboxylated groups of the two glutamate residues, Glu205 and Glu206.
4. The triazolopiperazine group collides with the phenyl group of residue Phe357
Constrained phenylethylamine compounds
Researchers at Abbott Laboratories identified three novel series of DPP-4 inhibitors using HTS. After more research and optimization ABT-341 was discovered (Figure 8). It is a potent and selective DPP-4 inhibitor with a 2D-structure very similar to sitagliptin. However, the 3D-structure is quite different. ABT-341 also has a trifluorophenyl group that occupies the S1-pocket and the free amino group, but the two carbonyl groups are orientated 180° away from each other. ABT-341 is also believed to interact with the Tyr547, probably because of steric hindrance between the cyclohexenyl ring and the tyrosine side-chain. Omarigliptin is one of such compound which is in Phase-III development by Merck & Co.
Pyrrolidine compounds
The pyrrolidine type of DPP-4 inhibitors was first discovered after HTS. Research showed that the pyrrolidine rings were the part of the compounds that fit into the binding site. Further development has led to fluoro substituted pyrrolidines that show superior activity, as well as pyrrolidines with fused cyclopropylrings that are highly active.
Xanthine-based compounds
This is a different class of inhibitors that was identified with HTS. Aromatic heterocyclic-based DPP-4 inhibitors have gained increased attention recently. The first patents describing xanthines (Figure 10) as DPP-4 inhibitors came from Boehringer-Ingelheim(BI) and Novo Nordisk. When xanthine based DPP-4 inhibitors are compared with sitagliptin and vildagliptin it has shown a superior profile. Xanthines are believed to have higher potency, longer-lasting inhibition and longer-lasting improvement of glucose tolerance.
Alogliptin

Alogliptin (Figure 9) is a novel DPP-4 inhibitor developed by the Takeda Pharmaceutical Company. Researchers hypothesized that a quinazolinone based structure (Figure 9) would have the necessary groups to interact with the active site on the DPP-4 complex. Quinazolinone based compounds interacted effectively with the DPP-4 complex, but suffered from low metabolic half-life. It was found that when replacing the quinazolinone with a pyrimidinedione, the metabolic stability was increased and the result was a potent, selective, bioavailable DPP-4 inhibitor named alogliptin. The quinazoline based compounds showed potent inhibition and excellent selectivity over related protease, DPP-8. However, short metabolic half-life due to oxidation of the A-ring phenyl group was problematic. At first, the researchers tried to make a fluorinated derivative. The derivative showed improved metabolic stability and excellent inhibition of the DPP-4 enzyme. However, it was also found to inhibit CYP 450 3A4 and block the hERG channel. The solution to this problem was to replace the quinazolinone with other heterocycles, but the quinazolinone could be replaced without any loss of DPP-4 inhibition. Alogliptin was discovered when quinazolinone was replaced with a pyrimidinedione. Alogliptin has shown excellent inhibition of DPP-4 and extraordinary selectivity, greater than 10.000 fold over the closely related serine proteases DPP-8 and DPP-9. Also, it does not inhibit the CYP 450 enzymes nor block the hERG channel at concentration up to 30 µM. Based on this data, alogliptin was chosen for preclinical evaluation. In January 2007 alogliptin was undergoing the phase III clinical trial and in October 2008 it was being reviewed by the U.S. Food and Drug Administration.
Researchers at BI discovered that using a buty-2-nyl group resulted in a potent candidate, called BI-1356 (Figure 10). In 2008 BI-1356 was undergoing phase III clinical trials; it was released as linagliptin in May 2011. X-ray crystallography has shown that that xanthine type binds the DPP-4 complex in a different way than other inhibitors:
1. The amino group also interacts with the Glu205, Glu206 and Tyr662
2. The buty-2-nyl group occupies the S1-pocket
3. The uracil group undergoes a π-stacking interaction with the Tyr547 residue
4. The quinazoline group undergoes a π-stacking interaction with the Trp629 residue
薬理学
薬剤 | 吸収 | 生物学的利用能 (%) | IC50 (nM) | 平均分布容積 (L) | タンパク質結合 (%) | 半減期 (時間,100 mg ドーズ) | 代謝 | 排泄 |
---|---|---|---|---|---|---|---|---|
シタグリプチン | 急速に吸収され、1~4時間で濃度がピークに達する | 87 | 18 | 198 | 38 | 12.4 | ごく一部はCYP 450 3A4および2C8を経由して肝代謝を受ける | 未変化体のまま尿中に排出される (79%) |
ビルダグリプチン | 急速に吸収され、1~2時間で濃度がピークに達する | 85 | 3 | 70.5 | 9.3 | 1.68(1日1回)、2.54(1日2回) | 加水分解により薬理学的に不活性な代謝物が生じる。一部(22%)は腎臓から未変化体として排泄される。 | 代謝物の排泄は尿(85%)と糞便(15%)を通じて行われる。 |
シタグリプチンおよびビルダグリプチンの薬物動態学的特性は、年齢、性別、またはBMIの影響を受けないようである。臨床研究では、シタグリプチンおよびビルダグリプチンには、2型糖尿病の治療後に起こりがちな副作用、例えば体重増加や高血糖がないことが示されている。感染症、咽頭痛、下痢などの副作用も認められている。