Vitamin B12/ja: Difference between revisions
Created page with "==摂取源== {{Anchor|Sources}} ===バクテリアと古細菌=== ビタミンB<sub>12</sub>は、自然界では特定の細菌と古細菌によって産生される。ヒトや他の動物では腸内細菌叢の一部の細菌によって合成されるが、ほとんどの栄養素の吸収が行われる小腸の下流にある大腸で作られるため、ヒトは..." Tags: Mobile edit Mobile web edit |
Created page with "他の哺乳類種(例:ウサギ、ナキウサギ、ビーバー、モルモット)は高繊維質の植物を摂取し、消化管を通過して盲腸と大腸で細菌発酵を受ける。この後腸発酵において、盲腸からの物質は「ネコトロープ」として排出され、再摂取される、この行..." Tags: Mobile edit Mobile web edit |
||
Line 140: | Line 140: | ||
ビタミンB<sub>12</sub>は、自然界では特定の[[bacteria/ja|細菌]]と[[archaea/ja|古細菌]]によって産生される。ヒトや他の動物では[[gut microbiota/ja|腸内細菌叢]]の一部の細菌によって合成されるが、ほとんどの栄養素の吸収が行われる[[small intestine/ja|小腸]]の下流にある[[large intestine/ja|大腸]]で作られるため、ヒトはこれを吸収できないと長い間考えられてきた。ウシやヒツジなどの[[ruminant/ja|反芻動物]]は前腸発酵動物であり、植物性食物は真の胃([[abomasum/ja|アボマサム]])に入る前に[[rumen/ja|ルーメン]]で微生物発酵を受けるため、細菌によって産生されたビタミンB<sub>12</sub>を吸収している。 | ビタミンB<sub>12</sub>は、自然界では特定の[[bacteria/ja|細菌]]と[[archaea/ja|古細菌]]によって産生される。ヒトや他の動物では[[gut microbiota/ja|腸内細菌叢]]の一部の細菌によって合成されるが、ほとんどの栄養素の吸収が行われる[[small intestine/ja|小腸]]の下流にある[[large intestine/ja|大腸]]で作られるため、ヒトはこれを吸収できないと長い間考えられてきた。ウシやヒツジなどの[[ruminant/ja|反芻動物]]は前腸発酵動物であり、植物性食物は真の胃([[abomasum/ja|アボマサム]])に入る前に[[rumen/ja|ルーメン]]で微生物発酵を受けるため、細菌によって産生されたビタミンB<sub>12</sub>を吸収している。 | ||
他の哺乳類種(例:[[rabbit/ja|ウサギ]]、[[pika/ja|ナキウサギ]]、[[beaver/ja|ビーバー]]、[[guinea pigs/ja|モルモット]])は高繊維質の植物を摂取し、消化管を通過して[[cecum/ja|盲腸]]と[[large intestine/ja|大腸]]で細菌発酵を受ける。この[[hindgut fermentation/ja|後腸発酵]]において、盲腸からの物質は「[[cecotrope/ja|ネコトロープ]]」として排出され、再摂取される、この行為は[[cecotrope/ja||子嚢菌]]と呼ばれる。再摂取によって、細菌発酵によって利用可能になった栄養素や、ビタミンB<sub>12</sub>を含む腸内細菌によって合成されたビタミンやその他の栄養素の吸収が可能になる。 | |||
<div lang="en" dir="ltr" class="mw-content-ltr"> | <div lang="en" dir="ltr" class="mw-content-ltr"> |
Revision as of 21:52, 5 April 2024
![]() コバラミン類の一般的な骨格式である。 | |
![]() | |
Clinical data | |
---|---|
Other names | Vitamin B12, vitamin B-12, cobalamin |
AHFS/Drugs.com | Monograph |
MedlinePlus | a605007 |
License data |
|
Routes of administration | 口から, 舌下, intravenous/ja (IV), intramuscular/ja (IM), 経鼻 |
ATC code | |
Legal status | |
Legal status |
|
Pharmacokinetic data | |
Bioavailability | 回腸の遠位半分で容易に吸収される。 |
Protein binding | 特定のトランスコバラミン血漿タンパク質への結合性が非常に高い。 ヒドロキソコバラミンの結合性はシアノコバラミンよりわずかに高い。 |
Metabolism | Liver/ja |
Elimination half-life | 約6日間 (肝臓では400日間) |
Excretion | Kidney/ja |
Identifiers | |
| |
CAS Number | |
PubChem CID | |
DrugBank | |
ChemSpider | |
UNII | |
KEGG | |
ChEMBL | |
Chemical and physical data | |
Formula | C63H88CoN14O14P |
Molar mass | 1355.388 g·mol−1 |
3D model (JSmol) | |
| |
|
ビタミンB12は、コバラミンとしても知られ、代謝に関与する水溶性のビタミンである。8種類のビタミンB群の1つである。DNA合成の補因子として、また脂肪酸とアミノ酸代謝の両方で使用する動物に必要である。神経系ではミエリンの合成、循環器系では骨髄における赤血球の成熟に重要な役割を果たす。植物はコバラミンを必要とせず、コバラミンに依存しない酵素で反応を行う。
ビタミンB12はすべてのビタミンの中で最も化学的に複雑であり、ヒトにとっては動物由来の食品またはサプリメントから摂取しなければならない唯一のビタミンである。ビタミンB12を合成できるのは一部の古細菌とバクテリアだけである。ビタミンB12欠乏症は、特に動物性食品の消費量が少ない集団に広く見られる症状である。これは、社会経済的地位の低さ、倫理的配慮、菜食主義などのライフスタイルの選択など、さまざまな理由によるものである。
ビタミンB12を含む食品には、肉、貝、肝臓、魚、鶏肉、卵、乳製品などがある。多くの朝食用シリアルはこのビタミンで食品強化されている。ビタミンB12欠乏症の治療と予防には、サプリメントや医薬品が利用できる。通常は口から摂取するが、欠乏症の治療には筋肉注射で投与することもある。
ビタミンB12欠乏は、妊娠中、幼児、高齢者に大きな影響を及ぼし、栄養失調のために中低開発国でより一般的である。先進国におけるビタミンB12欠乏の最も一般的な原因は、吸収が起こるためには食物由来のB12と結合していなければならない胃内在因子(IF)の損失による吸収障害である。第二の主な原因は、加齢に伴う胃酸産生の低下である(無胃酸症)。同じ理由で、プロトンポンプ阻害薬やH2遮断薬などの制酸剤を長期間使用している人はリスクが高くなる。
ベジタリアンや菜食主義者の食事では、栄養補助食品を摂取しない限り、十分なB12を摂取できないことがある。欠乏症は、手足の神経障害や、悪性貧血と呼ばれる血液疾患、赤血球が異常に大きくなる|貧血の一種と呼ばれる血液疾患によって特徴づけられることがある。その結果、疲労感、思考力の低下、ふらつき、息切れ、頻繁な感染症、食欲不振、手足の知覚異常、抑うつ、記憶喪失、錯乱、歩行困難、optic neuropathy/ja視神経障害、不可逆的な神経損傷、その他多くの症状が現れる。乳児の場合、欠乏を放置すると神経障害や貧血を引き起こす可能性がある。個体内の葉酸濃度は、ビタミンB12欠乏症の病理学的変化や症状の経過に影響を及ぼす可能性がある。妊婦におけるビタミンB12欠乏は、自然流産、神経管欠損症などの先天奇形、胎児の脳発達成長障害などのリスク上昇と強く関連している。
ビタミンB12は、ビタミンB12の欠乏により、血液中の赤血球の数が通常より少なくなる自己免疫疾患である悪性貧血の結果として発見された。ビタミンを吸収する能力は加齢とともに、特に60歳以上では低下する。
定義
ビタミンB12はコバルトの配位錯体で、コリン配位子の中心を占め、さらにベンズイミダゾール配位子とアデノシル基が結合している。多くの近縁種が知られており、これらは同様の挙動を示し、特にすべてがビタミンとして機能する。この化合物群は「コバラミン」と呼ばれることもある。これらの化合物は類似した分子構造を持ち、それぞれがビタミン欠乏の生体系でビタミン活性を示すことから、ビタマーと呼ばれる。ビタミンの活性は補酵素としてのもので、酵素触媒反応にその存在が必要であることを意味する。
- アデノシルコバラミン
- シアノコバラミンは、ビタミンB12のアデノシル配位子がシアン化物で置換されている。
- ヒドロキソコバラミンは、ビタミンB12のアデノシル配位子を水酸化物に置き換えたものである。
- ビタミンB12のアデノシル配位子はメチルで置換される。
シアノコバラミンは、B12の製造形態である。細菌発酵によってAdoB12とMeB12が生成され、亜硝酸ナトリウムと熱の存在下で青酸カリを加えることによってシアノコバラミンに変換される。シアノコバラミンが消費されると、生物学的に活性なAdoB12とMeB12に変換される。ビタミンB
12の2つの生理活性型は、サイトゾル中のメチルコバラミンとミトコンドリア中のアデノシルコバラミンである。
シアノコバラミンが栄養補助食品や食品強化に最もよく使われるのは、シアンが分解に対して分子を安定させるからである。メチルコバラミンも栄養補助食品として提供されている。ビタミンB12欠乏症の治療にアデノシルコバラミンやメチルコバラミン型を使用する利点はない。
ヒドロキソコバラミンは、ビタミンB12欠乏症を治療するために筋肉内に注射することができる。ヒドロキシル基がシアン化物によって置換され、尿中に排泄される無毒のシアノコバラミンが生成されるため、シアン化物中毒を治療する目的で静脈注射することもできる。
"疑似ビタミンB12"は、ビタミンに似た構造を持つがビタミン活性を持たないコリノイドである化合物を指す。偽ビタミンB12は、藻類の健康食品であるスピルリナに含まれるコリノイドの大部分である。
欠乏症
ビタミンB12欠乏症は、特に脳と神経系に深刻で不可逆的な損傷を引き起こす可能性がある。正常値よりわずかに低いレベルで欠乏すると、疲労、脱力感、ふらつき、めまい、息苦しさ、頭痛、口内炎、胃のむかつき、食欲低下などのさまざまな症状を引き起こす可能性がある、 歩行困難(ふらつきバランス障害)、筋力低下、抑うつ、記憶力低下、反射神経低下、錯乱、皮膚の青白さ、知覚異常など、特に60歳以上の人に多い。ビタミンB12欠乏症はまた、躁病や精神病の症状を引き起こすこともある。
ビタミンB12欠乏性貧血の主なタイプは悪性貧血であり、症状の三徴候を特徴とする:
- 骨髄前赤芽球癆を伴う貧血(巨赤芽球性貧血)。これはDNA合成(特にプリンとチミジン)の阻害によるものである。
- 消化器症状:軽度の下痢や便秘などの腸管運動の変化、膀胱や腸のコントロールができなくなる。これらは、細胞の入れ替わりが激しい組織部位において、DNA合成の欠陥が複製を阻害するためと考えられている。これは、悪性貧血における胃の壁細胞に対する自己免疫攻撃にも起因している可能性がある。胃前庭血管拡張症(スイカ胃と呼ばれることもある)と悪性貧血との関連がある。
- 神経学的症状:感覚または運動障害(反射の欠如、振動または軟部触覚の減退)および亜急性脊髄複合変性症がある。小児における欠損症状には、発達遅延、退行、過敏性、不随意運動、および筋緊張低下が含まれる。
ビタミンB12欠乏症は、最も一般的には吸収不良によって起こるが、摂取量の低下、免疫性胃炎、結合タンパク質の存在低下、特定の医薬品の使用によっても起こる。植物性食品にはビタミン欠乏症を予防するのに十分な量のビタミンが含まれていないため、菜食主義者-動物性食品を一切摂取しないことを選択した人々-にはリスクがある。また、菜食主義者-乳製品や卵などの動物性副産物は摂取するが、動物の肉は摂取しない-もリスクがある。ビタミンB12欠乏症は、ビタミンB12サプリメントを摂取していないか、ビタミン強化食品を摂取していないベジタリアン人口の40%から80%で観察されている。香港とインドでは、菜食主義者の約80%にビタミンB12欠乏症が認められている。ベジタリアンと同様に、菜食主義者も栄養補助食品を摂取するか、シリアル、植物性ミルク、栄養酵母などのビタミンB12強化食品を常食することでこれを避けることができる。高齢者は、加齢とともに胃酸の分泌が減少する傾向があり、無胃酸症として知られる状態になるため、リスクが高くなる。
亜酸化窒素の過剰摂取や過剰使用は、ビタミンB12の活性な一価型を不活性な二価型に変換する。
妊娠期、授乳期、幼児期
米国の推奨食事許容量 (RDA)は、妊娠期は2.6 μg/d、授乳期は2.8 µg/日である。これらの値の決定は、非妊娠女性のRDATemplate:値に、妊娠中に胎児に移行する分と母乳中に移行する分を加えたものである。しかし、同じ科学的根拠から、欧州食品安全機関(EFSA)は適切摂取量(AI)を、妊娠期は4.5 μg/d、授乳期は5.0 μg/dとしている。妊娠中、胎盤はB12を濃縮するので、新生児の血清濃度は母親よりも高くなる。より効果的に胎盤に到達するのは最近吸収されたビタミンであるため、母親になる女性が摂取するビタミンは、肝臓組織に含まれるビタミンよりも重要である。
動物性食品をほとんど摂取していない女性、あるいはベジタリアンやビーガンの女性は、動物性食品を多く摂取している女性よりも、妊娠中にビタミン欠乏症になるリスクが高い。この欠乏は貧血を引き起こし、母乳で育てられた乳児がビタミン欠乏症になるリスクも高くなる。ビタミンB12は、世界保健機関(WHO)が妊娠中の健康な女性に推奨するサプリメントのひとつではないが、特にベジタリアンやビーガンの食生活を送っている妊娠中の母親には、葉酸と一緒にマルチビタミンにビタミンB12を配合することがよく勧められている。
母乳中のビタミン濃度が低いのは、社会経済的地位が低いか、動物性食品の消費量が少ない家庭である。小麦粉やトウモロコシ粉の食品強化プログラムが義務づけられているのは、アフリカを中心とした数カ国だけで、インドには任意の強化プログラムがある。授乳中の母親が摂取するものは、肝組織の含有量よりも、最近吸収されたビタミンがより効果的に母乳に到達することの方が重要である。母乳中のビタミンB12は、栄養状態の良い母親でもビタミン欠乏症の母親でも、授乳期間中に減少する。6ヵ月を超える母乳育児は、授乳中の乳児の血清ビタミン状態が低いことを示す強い指標となる。特に、妊娠中にビタミン状態が不良であった場合や、母乳育児中の乳児に与える初期導入食品がビーガンであった場合に当てはまる。
離乳後の食事に動物性食品が少ないと、欠乏症のリスクは続く。乳幼児のビタミン低下には、貧血、発育不良、神経発達の遅れなどがある。血清B12が低値と診断された子どもは、筋肉注射で治療した後、経口栄養補助食品に移行することができる。
胃バイパス手術
病的肥満の治療には、さまざまな胃バイパス手術や胃制限手術が行われている。ルークス-エン-Y胃バイパス手術(RYGB)ではビタミンB12欠乏症のリスクが高まるが、スリーブ胃バイパス手術や胃バンディング手術ではビタミンB12欠乏症のリスクは高まらない。術後の経口補給では、ビタミン欠乏症を予防するために1000 μg/dが必要となることがある。
診断
ある総説によれば 現在のところ、ビタミンB12欠乏症の診断のための "ゴールドスタンダード"となる検査は存在しない。ビタミン欠乏症は、通常、ルーチンの全血球計算で平均赤血球量(MCV)の上昇を伴う貧血が認められた場合に疑われる。また、末梢血塗抹標本では、巨赤芽球や過分化した多形核白血球がみられることがある。診断は、ビタミンB12の血中濃度が成人で150~180pmol/L(200~250pg/mL)未満であることに基づく。しかし、組織のB12貯蔵量が枯渇しつつある一方で、血清値は維持できる。したがって、欠乏のカットオフポイントを超える血清B12値は、必ずしも十分なB12の状態を確認するものではない。このため、血中B12濃度だけに頼るよりも、血清ホモシステインが15μmol/L以上、メチルマロン酸(MMA)が0.271μmol/L以上上昇している方が、B12欠乏の指標として優れていると考えられている。しかし、MMAの上昇はB12欠乏症の人だけでなく、腎不全のある高齢者にも見られるため決定的なものではなく、ホモシステインの上昇は葉酸欠乏症の人にも見られるため決定的なものではない。さらに、メチルマロン酸値の上昇は、メチルマロン酸血症などの代謝障害に関連している可能性もある。神経系の障害があり、血液検査で結論が出ない場合は、腰椎穿刺を行って脳脊髄液を測定することがある。B12値を測定する。
血清ハプトコリンは循環B12の80-90%と結合し、トランスコバラミンIIによる細胞内運搬が不可能になる。これは循環貯蔵機能であると推測されている。生命を脅かすような重篤な疾患の中には、血清ビタミンB12が異常に高いために血清HCが上昇するものがあるが、同時にビタミンを細胞に運搬するトランスコバラミンIIに結合するビタミンが不十分であるために、症状のあるビタミン欠乏症として現れる可能性がある。
医療用途

欠乏症の治療
重度のビタミンB12欠乏症は、まず1000 μgの毎日の筋肉内注射で改善され、その後、毎月同量の注射または1000 μgの毎日の経口投与で維持される。通常のトランスポータータンパク質を介した吸収がなく、非常に非効率的な腸管受動吸収しか残らないため、1日の投与量はビタミンの必要量をはるかに超える。注射の副作用には、皮膚の発疹、かゆみ、悪寒、発熱、ほてり、吐き気、めまいなどがある。経口維持療法はこの問題を回避し、治療費を大幅に削減する。
シアン中毒
シアン化合物中毒に対しては、大量のヒドロキソコバラミンが静脈内に投与されることがあり、時にはチオ硫酸ナトリウムと併用されることもある。
食事に関する推奨事項
アメリカやイギリスでは、ほとんどの人が十分なビタミンB12を摂取しているという調査結果もある。しかし、他の研究によると、ビタミンB12が低いか、あるいは限界レベルである人の割合は、西洋世界では40%にも達する。穀物ベースの食品は、ビタミンを添加することによって強化することができる。ビタミンB12のサプリメントは、単一または複数のビタミン錠剤として入手可能である。ビタミンB12の医薬品製剤は、筋肉注射で投与することができる。動物性以外のビタミン源はほとんどないため、菜食主義者はB12摂取のために栄養補助食品や強化食品を摂取するか、深刻な健康被害を被るリスクを負うことを勧められる。発展途上国のある地域の子供たちは、動物性食品の少ない食事と相まって成長期に必要量が増加するため、特に危険である。
米国医学アカデミーは1998年にビタミンB12の推定平均必要量(EAR)と推奨食事許容量(RDA)を更新した。14歳以上の女性と男性のビタミンB12のEARは2.0 μg/日であり、RDAは2.4 μg/日である。RDAはEARより高いが、これは平均より必要量の多い人をカバーする量を特定するためである。妊娠中のRDAは2.6 μg/dayである。授乳期のRDAは2.8 μg/dに等しい。12ヵ月までの乳児の場合、適切な摂取量(AI)は0.4~0.5 μg/dayである(AIは、EARとRDAを決定するのに十分な情報がない場合に設定される)。1~13歳の子供の場合、RDAは年齢とともに0.9~1.8 μg/dayと増加する。高齢者の10~30%は食品に含まれるビタミンB12を効果的に吸収できない可能性があるため、50歳以上の高齢者は主にビタミンB12を強化した食品やビタミンB12を含むサプリメントを摂取することでRDAを満たすことが望ましい。安全性に関しては、十分なエビデンスがある場合には、ビタミンやミネラルについて耐容上限摂取量(ULと呼ばれる)が設定されている。ビタミンB12の場合、高用量摂取による有害作用に関するヒトでのデータがないため、ULは設定されていない。EAR、RDA、AI、ULを総称して食事摂取基準量(DRI)と呼ぶ。
欧州食品安全機関(EFSA)は、RDAの代わりに人口基準摂取量(PRI)、EARの代わりに平均必要量を用いて、一連の情報の集合体を「食事摂取基準値」と呼んでいる。AIとULは、EFSAによって米国と同じように定義されている。18歳以上の女性と男性については、十分摂取量(AI)は4.0 μg/日とされている。妊娠中のAIは4.5μg/日、授乳期は5.0 μg/日である。1~14歳の小児については、年齢とともにAIは1.5~3.5 μg/日と増加する。これらの基準値は米国のRDAよりも高い。EFSAは安全性についても検討し、ビタミンB12のULを設定するには十分な証拠がないという、米国と同じ結論に達した。
国立健康・栄養研究所は、12歳以上の推奨摂取量を2.4 μg/日と定めている。また、世界保健機関(WHO)もこのビタミンの成人推奨栄養摂取量として2.4 μg/日を用いている。
米国の食品および栄養補助食品の表示目的では、1食あたりの量は「1日当たりの価値の割合」(%DV)で表される。ビタミンB12の表示目的では、1日価値の100%は6.0 μgであったが、2016年5月27日に2.4 μgに下方修正された。更新された表示規制への適合は、年間食品売上高がUS$10 百万ドル以上の製造業者については2020年1月1日までに、それ以下の製造業者については2021年1月1日までに義務付けられた。新旧の成人一日摂取量の表は基準一日摂取量に掲載されている。
摂取源
バクテリアと古細菌
ビタミンB12は、自然界では特定の細菌と古細菌によって産生される。ヒトや他の動物では腸内細菌叢の一部の細菌によって合成されるが、ほとんどの栄養素の吸収が行われる小腸の下流にある大腸で作られるため、ヒトはこれを吸収できないと長い間考えられてきた。ウシやヒツジなどの反芻動物は前腸発酵動物であり、植物性食物は真の胃(アボマサム)に入る前にルーメンで微生物発酵を受けるため、細菌によって産生されたビタミンB12を吸収している。
他の哺乳類種(例:ウサギ、ナキウサギ、ビーバー、モルモット)は高繊維質の植物を摂取し、消化管を通過して盲腸と大腸で細菌発酵を受ける。この後腸発酵において、盲腸からの物質は「ネコトロープ」として排出され、再摂取される、この行為は|子嚢菌と呼ばれる。再摂取によって、細菌発酵によって利用可能になった栄養素や、ビタミンB12を含む腸内細菌によって合成されたビタミンやその他の栄養素の吸収が可能になる。
Non-ruminant, non-hindgut herbivores may have an enlarged forestomach and/or small intestine to provide a place for bacterial fermentation and B-vitamin production, including B12. For gut bacteria to produce vitamin B12, the animal must consume sufficient amounts of cobalt. Soil that is deficient in cobalt may result in B12 deficiency, and B12 injections or cobalt supplementation may be required for livestock.
Animal-derived foods
Animals store vitamin B12 from their diets in their livers and muscles and some pass the vitamin into their eggs and milk. Meat, liver, eggs and milk are therefore sources of the vitamin for other animals, including humans. Insects are a source of B12 for animals (including other insects and humans). Animal-derived food sources with a high concentration of vitamin B12 include liver and other organ meats from lamb, veal, beef, and turkey; also shellfish and crab meat.
Plants and algae
There is some evidence that bacterial fermentation of plant foods and symbiotic relationships between algae and bacteria can provide vitamin B12. However, the Academy of Nutrition and Dietetics considers plant and algae sources "unreliable", stating that vegans should turn to fortified foods and supplements instead.
Natural plant and algae sources of vitamin B12 include fermented plant foods such as tempeh and seaweed-derived foods such as nori and laverbread. Methylcobalamin has been identified in Chlorella vulgaris. Since only bacteria and some archea possess the genes and enzymes necessary to synthesize vitamin B12, plant and algae sources all obtain the vitamin secondarily from symbiosis with various species of bacteria, or in the case of fermented plant foods, from bacterial fermentation.
Fortified foods
Foods for which vitamin B12-fortified versions are available include breakfast cereals, plant-derived milk substitutes such as soy milk and oat milk, energy bars, and nutritional yeast. The fortification ingredient is cyanocobalamin. Microbial fermentation yields adenosylcobalamin, which is then converted to cyanocobalamin by addition of potassium cyanide or thiocyanate in the presence of sodium nitrite and heat.
As of 2019, nineteen countries require food fortification of wheat flour, maize flour or rice with vitamin B12. Most of these are in southeast Africa or Central America.
Vegan advocacy organizations, among others, recommend that every vegan consume B12 from either fortified foods or supplements.
Supplements

Vitamin B12 is included in multivitamin pills; in some countries grain-based foods such as bread and pasta are fortified with B12. In the US, non-prescription products can be purchased providing up to 5,000 µg each, and it is a common ingredient in energy drinks and energy shots, usually at many times the recommended dietary allowance of B12. The vitamin can also be supplied on prescription and delivered via injection or other means.
Sublingual methylcobalamin, which contains no cyanide, is available in 5 mg tablets. The metabolic fate and biological distribution of methylcobalamin are expected to be similar to that of other sources of vitamin B12 in the diet. The amount of cyanide in cyanocobalamin is generally not a concern, even in the 1,000 µg dose, since the amount of cyanide there (20 µg in a 1,000 µg cyanocobalamin tablet) is less than the daily consumption of cyanide from food, and therefore cyanocobalamin is not considered a health risk.
Intramuscular or intravenous injection
Injection of hydroxycobalamin is often used if digestive absorption is impaired, but this course of action may not be necessary with high-dose oral supplements (such as 0.5–1.0 mg or more), because with large quantities of the vitamin taken orally, even the 1% to 5% of free crystalline B12 that is absorbed along the entire intestine by passive diffusion may be sufficient to provide a necessary amount.
A person with cobalamin C disease (which results in combined methylmalonic aciduria and homocystinuria) may require treatment with intravenous or intramuscular hydroxocobalamin or transdermal B12, because oral cyanocobalamin is inadequate in the treatment of cobalamin C disease.
Nanotechnologies used in vitamin B12 supplementation
Conventional administration does not ensure specific distribution and controlled release of vitamin B12. Moreover, therapeutic protocols involving injection require health care people and commuting of patients to the hospital thus increasing the cost of the treatment and impairing the lifestyle of patients. Targeted delivery of vitamin B12 is a major focus of modern prescriptions. For example, conveying the vitamin to the bone marrow and nerve cells would help myelin recovery. Currently, several nanocarriers strategies are being developed for improving vitamin B12 delivery with the aim to simplify administration, reduce costs, improve pharmacokinetics, and ameliorate the quality of patients' lives.
Pseudovitamin-B12
Pseudovitamin-B12 refers to B12-like analogues that are biologically inactive in humans. Most cyanobacteria, including Spirulina, and some algae, such as Porphyra tenera (used to make a dried seaweed food called nori in Japan), have been found to contain mostly pseudovitamin-B12 instead of biologically active B12. These pseudo-vitamin compounds can be found in some types of shellfish, in edible insects, and at times as metabolic breakdown products of cyanocobalamin added to dietary supplements and fortified foods.
Pseudovitamin-B12 can show up as biologically active vitamin B12 when a microbiological assay with Lactobacillus delbrueckii subsp. lactis is used, as the bacteria can utilize the pseudovitamin despite it being unavailable to humans. To get a reliable reading of B12 content, more advanced techniques are available. One such technique involves pre-separation by silica gel and then assessment with B12-dependent E. coli bacteria.
A related concept is antivitamin B12, compounds (often synthetic B12 analogues) that not only have no vitamin action, but also actively interfere with the activity of true vitamin B12. The design of these compounds mainly involve replacement of the metal ion with rhodium, nickel, or zinc; or the attachment of an inactive ligand such as 4-ethylphenyl. These compounds have the potential to be used for analyzing B12 utilization pathways or even attacking B12-dependent pathogens.
Drug interactions
H2-receptor antagonists and proton-pump inhibitors
Gastric acid is needed to release vitamin B12 from protein for absorption. Reduced secretion of gastric acid and pepsin, from the use of H2 blocker or proton-pump inhibitor (PPI) drugs, can reduce absorption of protein-bound (dietary) vitamin B12, although not of supplemental vitamin B12. H2-receptor antagonist examples include cimetidine, famotidine, nizatidine, and ranitidine. PPIs examples include omeprazole, lansoprazole, rabeprazole, pantoprazole, and esomeprazole. Clinically significant vitamin B12 deficiency and megaloblastic anemia are unlikely, unless these drug therapies are prolonged for two or more years, or if in addition the person's dietary intake is below recommended levels. Symptomatic vitamin deficiency is more likely if the person is rendered achlorhydric (a complete absence of gastric acid secretion), which occurs more frequently with proton pump inhibitors than H2 blockers.
Metformin
Reduced serum levels of vitamin B12 occur in up to 30% of people taking long-term anti-diabetic metformin. Deficiency does not develop if dietary intake of vitamin B12 is adequate or prophylactic B12 supplementation is given. If the deficiency is detected, metformin can be continued while the deficiency is corrected with B12 supplements.
Other drugs
Certain medications can decrease the absorption of orally consumed vitamin B12, including colchicine, extended-release potassium products, and antibiotics such as gentamicin, neomycin and tobramycin. Anti-seizure medications phenobarbital, pregabalin, primidone and topiramate are associated with lower than normal serum vitamin concentration. However, serum levels were higher in people prescribed valproate. In addition, certain drugs may interfere with laboratory tests for the vitamin, such as amoxicillin, erythromycin, methotrexate and pyrimethamine.
Chemistry
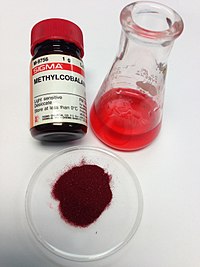
Vitamin B12 is the most chemically complex of all the vitamins. The structure of B12 is based on a corrin ring, which is similar to the porphyrin ring found in heme. The central metal ion is cobalt. As isolated as an air-stable solid and available commercially, cobalt in vitamin B12 (cyanocobalamin and other vitamers) is present in its +3 oxidation state. Biochemically, the cobalt center can take part in both two-electron and one-electron reductive processes to access the "reduced" (B12r, +2 oxidation state) and "super-reduced" (B12s, +1 oxidation state) forms. The ability to shuttle between the +1, +2, and +3 oxidation states is responsible for the versatile chemistry of vitamin B12, allowing it to serve as a donor of deoxyadenosyl radical (radical alkyl source) and as a methyl cation equivalent (electrophilic alkyl source).
Four of the six coordination sites are provided by the corrin ring, and a fifth by a dimethylbenzimidazole group. The sixth coordination site, the reactive center, is variable, being a cyano group (–CN), a hydroxyl group (–OH), a methyl group (–CH3) or a 5′-deoxyadenosyl group. Historically, the covalent carbon–cobalt bond is one of the first examples of carbon–metal bonds to be discovered in biology. The hydrogenases and, by necessity, enzymes associated with cobalt utilization, involve metal–carbon bonds. Animals have the ability to convert cyanocobalamin and hydroxocobalamin to the bioactive forms adenosylcobalamin and methylcobalamin by means of enzymatically replacing the cyano or hydroxyl groups.

Methods for the analysis of vitamin B12 in food
Several methods have been used to determine the vitamin B12 content in foods including microbiological assays, chemiluminescence assays, polarographic, spectrophotometric and high-performance liquid chromatography processes. The microbiological assay has been the most commonly used assay technique for foods, utilizing certain vitamin B12-requiring microorganisms, such as Lactobacillus delbrueckii subsp. lactis ATCC7830. However, it is no longer the reference method due to the high measurement uncertainty of vitamin B12.
Furthermore, this assay requires overnight incubation and may give false results if any inactive vitamin B12 analogues are present in the foods. Currently, radioisotope dilution assay (RIDA) with labelled vitamin B12 and hog IF (pigs) have been used to determine vitamin B12 content in food. Previous reports have suggested that the RIDA method is able to detect higher concentrations of vitamin B12 in foods compared to the microbiological assay method.
Biochemistry
Coenzyme function
Vitamin B12 functions as a coenzyme, meaning that its presence is required in some enzyme-catalyzed reactions. Listed here are the three classes of enzymes that sometimes require B12 to function (in animals):
- Isomerases
- Rearrangements in which a hydrogen atom is directly transferred between two adjacent atoms with concomitant exchange of the second substituent, X, which may be a carbon atom with substituents, an oxygen atom of an alcohol, or an amine. These use the adoB12 (adenosylcobalamin) form of the vitamin.
- Methyltransferases
- Methyl (–CH3) group transfers between two molecules. These use the MeB12 (methylcobalamin) form of the vitamin.
- Dehalogenases
- Some species of anaerobic bacteria synthesize B12-dependent dehalogenases, which have potential commercial applications for degrading chlorinated pollutants. The microorganisms may either be capable of de novo corrinoid biosynthesis or are dependent on exogenous vitamin B12.
In humans, two major coenzyme B12-dependent enzyme families corresponding to the first two reaction types, are known. These are typified by the following two enzymes:

Methylmalonyl coenzyme A mutase (MUT) is an isomerase enzyme which uses the AdoB12 form and reaction type 1 to convert L-methylmalonyl-CoA to succinyl-CoA, an important step in the catabolic breakdown of some amino acids into succinyl-CoA, which then enters energy production via the citric acid cycle. This functionality is lost in vitamin B12 deficiency, and can be measured clinically as an increased serum methylmalonic acid (MMA) concentration. The MUT function is necessary for proper myelin synthesis. Based on animal research, it is thought that the increased methylmalonyl-CoA hydrolyzes to form methylmalonate (methylmalonic acid), a neurotoxic dicarboxylic acid, causing neurological deterioration.
Methionine synthase, coded by MTR gene, is a methyltransferase enzyme which uses the MeB12 and reaction type 2 to transfer a methyl group from 5-methyltetrahydrofolate to homocysteine, thereby generating tetrahydrofolate (THF) and methionine. This functionality is lost in vitamin B12 deficiency, resulting in an increased homocysteine level and the trapping of folate as 5-methyl-tetrahydrofolate, from which THF (the active form of folate) cannot be recovered. THF plays an important role in DNA synthesis, so reduced availability of THF results in ineffective production of cells with rapid turnover, in particular red blood cells, and also intestinal wall cells which are responsible for absorption. THF may be regenerated via MTR or may be obtained from fresh folate in the diet. Thus all of the DNA synthetic effects of B12 deficiency, including the megaloblastic anemia of pernicious anemia, resolve if sufficient dietary folate is present. Thus the best-known "function" of B12 (that which is involved with DNA synthesis, cell-division, and anemia) is actually a facultative function which is mediated by B12-conservation of an active form of folate which is needed for efficient DNA production. Other cobalamin-requiring methyltransferase enzymes are also known in bacteria, such as Me-H4-MPT, coenzyme M methyltransferase.
Physiology
Absorption
Vitamin B12 is absorbed by a B12-specific transport proteins or via passive diffusion. Transport-mediated absorption and tissue delivery is a complex process involving three transport proteins: haptocorrin (HC), intrinsic factor (IF) and transcobalamin II (TC2), and respective membrane receptor proteins. HC is present in saliva. As vitamin-containing food is digested by hydrochloric acid and pepsin secreted into the stomach, HC binds the vitamin and protected it from acidic degradation. Upon leaving the stomach the hydrochloric acid of the chyme is neutralized in the duodenum by bicarbonate, and pancreatic proteases release the vitamin from HC, making it available to be bound by IF, which is a protein secreted by gastric parietal cells in response to the presence of food in the stomach. IF delivers the vitamin to receptor proteins cubilin and amnionless, which together form the cubam receptor in the distal ileum. The receptor is specific to the IF-B12 complex, and so will not bind to any vitamin content that is not bound to IF.
Investigations into the intestinal absorption of B12 confirm that the upper limit of absorption per single oral dose is about 1.5 µg, with 50% efficiency. In contrast, the passive diffusion process of B12 absorption — normally a very small portion of total absorption of the vitamin from food consumption — may exceed the haptocorrin- and IF-mediated absorption when oral doses of B12 are very large, with roughly 1% efficiency. Thus, dietary supplement B12 supplementation at 500 to 1000 µg per day allows pernicious anemia and certain other defects in B12 absorption to be treated with daily oral megadoses of B12 without any correction of the underlying absorption defects.
After the IF/B12 complex binds to cubam the complex is disassociated and the free vitamin is transported into the portal circulation. The vitamin is then transferred to TC2, which serves as the circulating plasma transporter, Hereditary defects in production of TC2 and its receptor may produce functional deficiencies in B12 and infantile megaloblastic anemia, and abnormal B12 related biochemistry, even in some cases with normal blood B12 levels. For the vitamin to serve inside cells, the TC2-B12 complex must bind to a cell receptor protein and be endocytosed. TC2 is degraded within a lysosome, and free B12 is released into the cytoplasm, where it is transformed into the bioactive coenzyme by cellular enzymes.
Malabsorption
Antacid drugs that neutralize stomach acid and drugs that block acid production (such as proton-pump inhibitors) will inhibit absorption of B12 by preventing release from food in the stomach. Other causes of B12 malabsorption include intrinsic factor deficiency, pernicious anemia, bariatric surgery pancreatic insufficiency, obstructive jaundice, tropical sprue and celiac disease, and radiation enteritis of the distal ileum. Age can be a factor. Elderly people are often achlorhydric due to reduced stomach parietal cell function, and thus have an increased risk of B12 deficiency.
Storage and excretion
How fast B12 levels change depends on the balance between how much B12 is obtained from the diet, how much is secreted and how much is absorbed. The total amount of vitamin B12 stored in the body is about 2–5 mg in adults. Around 50% of this is stored in the liver. Approximately 0.1% of this is lost per day by secretions into the gut, as not all these secretions are reabsorbed. Bile is the main form of B12 excretion; most of the B12 secreted in the bile is recycled via enterohepatic circulation. Excess B12 beyond the blood's binding capacity is typically excreted in urine. Owing to the extremely efficient enterohepatic circulation of B12, the liver can store 3 to 5 years' worth of vitamin B12; therefore, nutritional deficiency of this vitamin is rare in adults in the absence of malabsorption disorders. In the absence of enterohepatic reabsorption, only months to a year of vitamin B12 are stored.
Cellular reprogramming
Vitamin B12 through its involvement in one-carbon metabolism plays a key role in cellular reprogramming and tissue regeneration and epigenetic regulation. Cellular reprogramming is the process by which somatic cells can be converted to a pluripotent state. Vitamin B12 levels affect the histone modification H3K36me3, which suppresses illegitimate transcription outside of gene promoters. Mice undergoing in vivo reprogramming were found to become depleted in B12 and show signs of methionine starvation while supplementing reprogramming mice and cells with B12 increased reprogramming efficiency, indicating a cell-intrinsic effect.
Synthesis
Biosynthesis
Vitamin B12 is derived from a tetrapyrrolic structural framework created by the enzymes deaminase and cosynthetase which transform aminolevulinic acid via porphobilinogen and hydroxymethylbilane to uroporphyrinogen III. The latter is the first macrocyclic intermediate common to heme, chlorophyll, siroheme and B12 itself. Later steps, especially the incorporation of the additional methyl groups of its structure, were investigated using 13C methyl-labelled S-adenosyl methionine. It was not until a genetically engineered strain of Pseudomonas denitrificans was used, in which eight of the genes involved in the biosynthesis of the vitamin had been overexpressed, that the complete sequence of methylation and other steps could be determined, thus fully establishing all the intermediates in the pathway.
Species from the following genera and the following individual species are known to synthesize B12: Propionibacterium shermanii, Pseudomonas denitrificans, Streptomyces griseus, Acetobacterium, Aerobacter, Agrobacterium, Alcaligenes, Azotobacter, Bacillus, Clostridium, Corynebacterium, Flavobacterium, Lactobacillus, Micromonospora, Mycobacterium, Nocardia, Proteus, Rhizobium, Salmonella, Serratia, Streptococcus and Xanthomonas.
工業用
ビタミンB12の工業的生産は、選ばれた微生物の発酵によって達成される。かつて真菌であると考えられていたストレプトマイセス・グリセウスは、長年ビタミンB12の商業的供給源であった。今日では、Pseudomonas denitrificans'とPropionibacterium freudenreichii'の亜種shermaniiがより一般的に使用されている。これらは収量を高めるために特別な条件下で栽培される。Rhone-Poulenc社はP. denitrificansを遺伝子操作することで収量を向上させた。プロピオニバクテリウムは、外毒素や内毒素を産生せず、アメリカの食品医薬品局から一般に安全と認められている(GRASステータスを付与されている)。
2008年のビタミンB12の世界総生産量は35,000 kg(77,175 ポンド)であった。
研究室
実験室での完全なB12の合成は1972年にロバート・バーンズ・ウッドワードとアルバート・エッシェンモーザーによって達成された。この仕事には、19カ国から91人の博士研究員(ほとんどがハーバード大学)と12人の博士課程の学生(チューリッヒ工科大学)が参加した。研究グループは既知の中間体であるコバリン酸を調製しただけであり、そのビタミンB12への化学変換は以前に報告されている。ビタミンB12のこの合成は、72の化学的工程を要し、全体の化学的収率が0.01%をはるかに下回るという長さのため、実用上の意味はない。1972年以来、散発的な合成の努力はあったが、Eschenmoser-Woodward合成は、完成された(正式な)唯一の全合成である。
歴史
欠乏症の記述
1849年から1887年にかけて、トーマス・アジソンが悪性貧血の症例を記述し、ウィリアム・オスラーとウィリアム・ガードナーが神経障害の症例を最初に記述し、ヘイムがこの病態における末梢血中の大きな赤血球について記述した、 彼はこれを「巨赤芽球」(現在では巨赤芽球と呼ぶ)と呼び、Paul Ehrlichは骨髄中の巨赤芽球を同定し、Ludwig Lichtheimは脊髄症の症例を記載した。
抗貧血食品としてのレバーの同定
1920年代、George Whippleは、生のレバーを大量に摂取することが、犬の失血性貧血を最も速やかに治癒させるようであることを発見し、レバーを食べることが悪性貧血を治療するかもしれないという仮説を立てた。エドウィン・コーンは、悪性貧血の治療に天然の肝臓製品の50倍から100倍の効力がある肝臓エキスを調製した。ウィリアム・キャッスルは、胃液に「内在因子」が含まれていることを証明し、この因子が肉の摂取と組み合わさると、悪性貧血の状態でもビタミンが吸収されることを示した。1934年、ジョージ・ウィップルはウィリアム・P・マーフィー、ジョージ・マイノットと共に、後にビタミンB12を多量に含むことが判明した濃縮肝臓を用いた悪性貧血の効果的な治療法の発見により、1934年のノーベル生理学・医学賞を受賞した。
活性化合物の同定
米国農務省酪農局に勤務していたMary Shaw Shorbは、ヨーグルトやその他の培養乳製品の製造に使用される細菌株Lactobacillus lactis Dorner(LLD)の研究を任された。LLDの培地には肝臓エキスが必要だった。Shorbは、同じ肝臓エキスが悪性貧血の治療に使われていることを知っており(彼女の義父はこの病気で亡くなっていた)、LLDを活性化合物を同定するためのアッセイ法として開発できると結論づけた。メリーランド大学在学中、彼女はメルク社から少額の助成金を受け、同社のKarl Folkersと共同でLLDアッセイ法を開発した。これによって「LLD因子」が細菌の増殖に不可欠であることが特定された。ショーブ、フォルカー、そしてケンブリッジ大学のアレクサンダー・R・トッドは、LLDアッセイを用いて肝臓抽出液から抗悪性貧血因子を抽出し、精製してビタミンB12と命名した。1955年、トッドはビタミンの構造解明に貢献した。分子の完全な化学構造はドロシー・ホジキンによって結晶学データに基づいて決定され、1955年に発表された。ホジキンはその後、インスリンの構造を解読した。
ジョージ・ウィップル、ジョージ・マイノット、ウィリアム・マーフィーは、ビタミンの研究で1934年にノーベル賞を受賞した。他にも、アレクサンダー・R・トッド(1957年)、ドロシー・ホジキン(1964年)、ロバート・バーンズ・ウッドワード(1965年)の3人のノーベル賞受賞者が、ビタミンの研究に重要な貢献をした。
商業生産
ビタミンB12の工業的生産は、選択された微生物の発酵によって達成される。上述のように、B12の完全合成は1972年にロバート・バーンズ・ウッドワードとアルバート・エッシェンモーザーによって達成されたが、このプロセスは70以上の工程を必要とし、収率も0.01%をはるかに下回るため、商業的な可能性はない。
社会と文化
1970年代、ボルチモア在住の医師ジョン・A・マイヤーズは、さまざまな病状に対してビタミンとミネラルを静脈注射するプログラムを開発した。その処方には1000 μgのシアノコバラミンが含まれていた。これはマイヤーズのカクテルとして知られるようになった。1984年にマイヤーズが亡くなった後、他の医師や自然療法家たちが、疲労、エネルギー低下、ストレス、不安、偏頭痛、うつ病、免疫不全、体重減少などを治療するために、根拠のない健康強調表示をした「微量栄養素点滴療法」を処方するようになった。しかし、症例研究の報告以外には、科学的文献で確認された効果はない。診療所やスパの開業医は、これらのビタミンB12を配合した点滴製剤を処方しているが、ビタミンB12だけの筋肉注射も行っている。メイヨークリニックのレビューでは、ビタミンB12注射がエネルギー増強や体重減少を助けるという確かな証拠はないと結論づけている。
高齢者では、医師が不適切なシアノコバラミン注射を繰り返し処方・投与しているという証拠がある。これは、ある大規模な研究において、被験者の大半が血清濃度が正常であったか、注射前の検査を受けていなかったことからも明らかである。
こちらも参照
さらに読む
- Gherasim C, Lofgren M, Banerjee R (May 2013). "Navigating the B(12) road: assimilation, delivery, and disorders of cobalamin". J. Biol. Chem. 288 (19): 13186–13193. doi:10.1074/jbc.R113.458810. PMC 3650358. PMID 23539619.
外部リンク
- Cyanocobalamin at the U.S. National Library of Medicine Medical Subject Headings (MeSH)